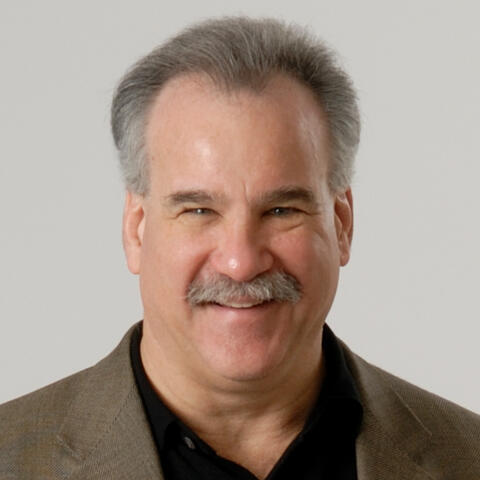
For 25 years, the Soai Reaction has endured as one of the most mysterious reactions in organic chemistry, but Reynold C. Fuson Professor of Chemistry Scott Denmark and graduate student Soumitra Athavale have shed new light on this strange chemical reaction that’s attracted the attention of scientists for decades.
First reported by Japanese chemist Kenso Soai in 1995, the reaction defies belief in multiple ways, and there is no other reaction like it in all of organic chemistry, Denmark said.
“Not surprisingly, a reaction this special had attracted a tremendous amount of attention, not only in the organic chemistry community but also in those disciplines interested in the origin of life and origin of biomolecular homochirality,” he said. Biomolecular homochirality is the phenomenon that molecules in the biosphere tend to be of a single handedness, like D-sugars and L-amino acids.
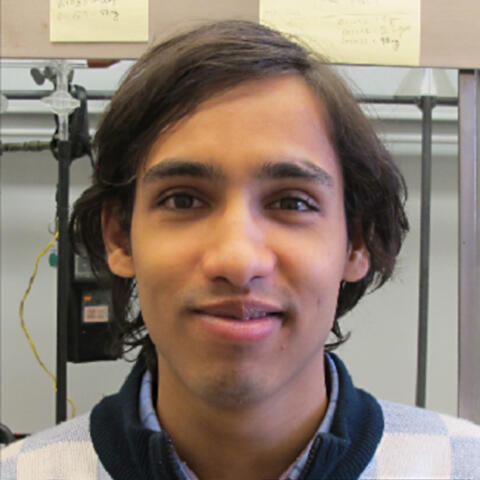
Understanding this special reaction could help explain this phenomenon that biomolecules “exist almost exclusively in one mirror image form,” according to an article in Chemistry World (the news magazine of the Royal Society of Chemistry) discussing the Denmark Group’s research, which was recently published in Nature Chemistry.
Their research reveals that “an enzyme-like mechanism allows tiny chiral imbalances to create single enantiomer products from non-chiral starting materials,” according to Chemistry World.
The Soai reaction, Denmark explained, is an excellent example of three phenomena in catalysis: autocatalysis, enantioselective catalysis and non-linear effects (asymmetric amplification).
Autocatalysis, he said, is especially important in frontier fields like systems chemistry and origins of life while enantioselective catalysis is a cornerstone of modern synthetic methods. The mechanistic understanding of the Soai Reaction will assist the design and discovery of new reactions that share these characteristics, Denmark said, and provide a platform for further studies and explorations in the fascinating area of asymmetric autocatalysis – a concept with foundational importance in chemistry.
Through the years, many researchers have studied various aspects of this reaction trying to understand its ability to perform spectacular feats of stereochemistry, the three-dimensional arrangement of atoms and molecules and their effect on chemical reactions. Those studies have resulted in some important mechanistic insights.
But, elusive aspects still remained, Denmark explained, including a holistic understanding of the origin of the unique catalyst structure; an explanation for the extremely narrow structural requirements for autocatalysis; and most importantly, a compelling transition state model that explains the basis for the formation of the homochiral product from this catalyst.
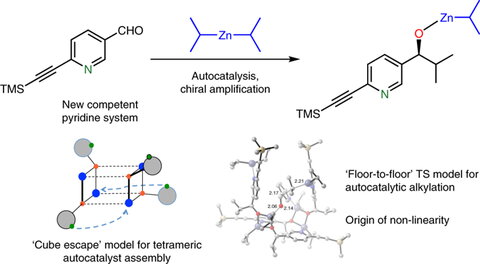
This research by the Denmark Group provides definitive answers to these mysteries as the research team explains:
“Through a combination of structural, mechanistic and computational investigations, and benefitting from the unprecedented discovery of amplifying autocatalysis in a related, pyridine system, they demonstrate how the tetrameric autocatalyst is assembled and operates. The homochiral tetramer is assembled by a pyridine assisted cube escape (see Figure). This cluster engages the substrate aldehyde through a two-point coordination to the unsaturated (alkoxy) zinc centers on the catalyst floor. In an enzyme-like fashion, alkylation occurs by preferred (by 4.2 kcal/mol) diisopropylzinc delivery from only one arm of the bound complex to produce the homochiral product – the (S)-autocatalyst generates the (S)-product – resulting in an enantioselective autocatalytic process. Critically, such a floor-to-floor binding mode is inaccessible to the energetically favored racemic tetramer, rendering it catalytically incompetent and establishing a structural basis for the dramatic asymmetric amplification (non-linearity).”
Denmark and Athavale collaborated in this research with Kendall N. Houk, a professor at the University of California at Los Angeles, and his graduate student Adam Simon, who performed the computational DFT studies.
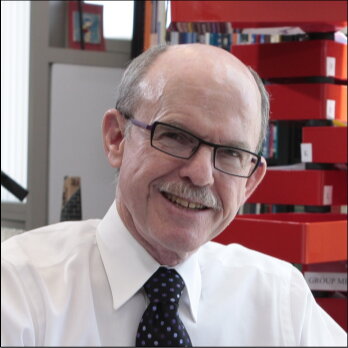
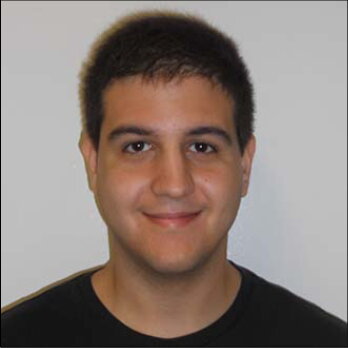