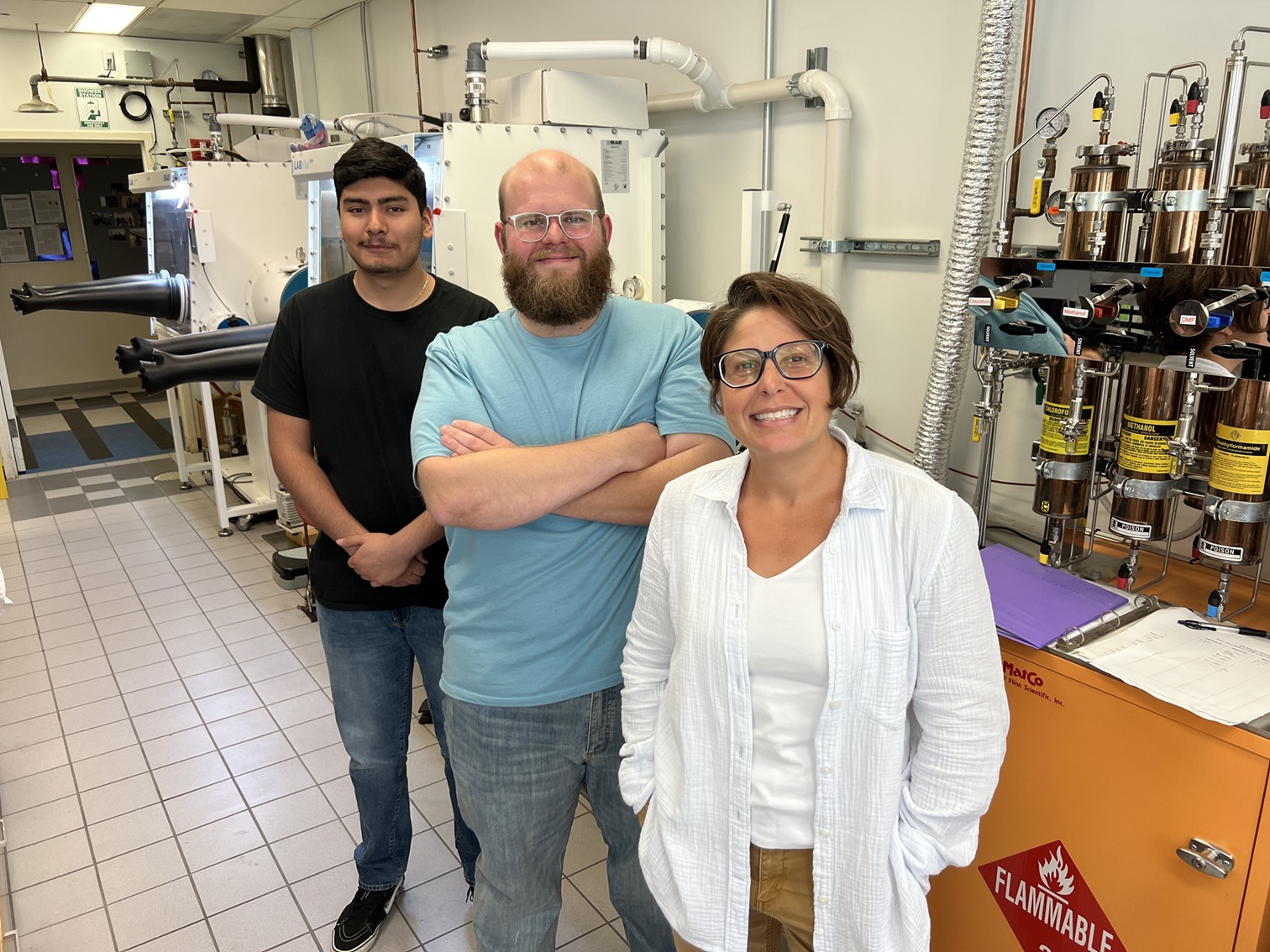
With a natural enzyme as inspiration, researchers at the University of Illinois Urbana-Champaign have designed an artificial enzyme that converts methanol to formaldehyde in a process that has the potential to massively reduce energy use in the commercial production of this prevalently used chemical.
The artificial metalloenzyme (ArM) created by chemistry professor Lisa Olshansky and graduate student Peter Thompson is easy to use, very stable, and functions at room temperature in water.
An ingredient in many materials and processes, including glues, resins, textiles, disinfectants, building materials, automobile parts, and labs, the production of formaldehyde from methanol exceeds 20 million tons annually and mostly via reactors operating at 500° to 900° Fahrenheit.
“Developing a biocatalytic system that can operate in water at room temperature has the potential to massively impact energy expenditures in this industrial sector,” Olshansky said.
The results of their study were recently published in the Proceedings of the National Academy of Sciences.
Thompson explained that some artificial metalloenzymes are incredibly sensitive to ambient pressures and air and need to be kept cold or air free, but this one is very stable in a range of conditions.
“Theoretically, you could leave it on the bench for a couple of hours, and it's not going to degrade and then be unusable. It's great. And we can prepare it on large scales without a ton of material, so that's another aspect that I think helps with our application goals,” Thompson said.
Artificial metalloenzymes have been around for since the early 70s but the field has been expanding rapidly in recent decades as scientific advancements in a few major classes of ArMs have given rise to a huge diversity of chemistry, including Frances Arnold’s 2018 Nobel Prize winning work evolving artificial metalloenzymes to realize new-to-nature chemical reactions.
The researchers said their ArM represents a whole new branch of this field.
“This is another protein scaffold that we are reporting that kind of joins the ranks among the other artificial metalloenzymes that have been created. And so, it's just cool to be the first to report that,” Thompson said.
Like the native alcohol dehydrogenase (ADH) enzymes, the artificial version they created is also dependent on lanthanum ions – a rare earth metal ion – to catalyze reactions.
More than two decades ago, scientists discovered that some bacteria use these rare earth dependent ADHs to meet their metabolic needs, which sparked scientific interest in how these enzymes work.
Curious about them in their previous work with switchable artificial metalloenzymes, the Olshansky lab initially set out a few years ago to create an artificial model for the purpose of learning more about their natural counterparts, whose study in a lab environment is challenging due to the unique biochemistry at play.
But as the work progressed on the artificial version and their scientific questions evolved, their efforts culminated in this rare-earth-dependent artificial metalloenzyme that mimics its natural counterpart in structure and function.
“In addition to mimicking the structure, it also does the same catalysis, the same chemistry,” Olshansky said.
This ArM catalyzes the conversion of benzyl alcohol to benzaldehyde like its native counterpart and provides researchers with a platform for further chemical and structural studies that could answer long-standing questions about the natural enzyme’s mechanism, structure, and function.
And their platform is primed for future mechanistic, biocatalytic, and biosensing applications. In addition to its potential industrial use for converting methanol to formaldehyde, the researchers hope to focus on a few other primary applications.
“One is using this ArM to bind other metals, including transition metals, to catalyze other reactions, like cross coupling reactions, which make carbon-carbon bonds, and expand into new-to-nature chemical reactions that aren't found in natural enzymes but can be very important to humankind, for example, making pharmaceuticals and new medicines,” Olshansky explained.
Thompson recalls the two most pivotal moments in their work. First was seeing the x-ray diffraction image of their protein scaffold – basically a photograph of a molecule – that finally confirmed the structure of their creation.
Their collaborators on the study, researchers in the lab of Professor Jennifer Bridwell-Rabb at the University of Michigan, handled the crystal x-ray diffraction.
Another pivotal moment was successfully incorporating the lanthanum into the scaffold and seeing actual chemical activity.
“This paper wouldn't have happened without the structure. So that was really exciting,” Thompson said. “And then seeing a couple pieces of evidence showing us that activity was happening and actually being able to see that in real time was really cool.”
Carlos Nava, an undergraduate researcher in the Olshansky lab, spent the summer working on strengthening bonds within their protein scaffold to make it more robust as they prepare to move forward with other new-to-nature reactions or improving reactivity.
Nava, who is a mentee of Thompson through the C2 program, said he really enjoyed doing research in the lab.
“I enjoy learning the spectroscopy, the data, everything,” he said.
Thompson said mentoring is one of the things he has enjoyed most about his PhD work.
Editor’s notes:
DOI: 10.1073/pnas.2405836121
Contact: Lisa Olshansky at lolshans@illinois.edu
This research was partly funded by a National Institutes of Health grant (GM138138).