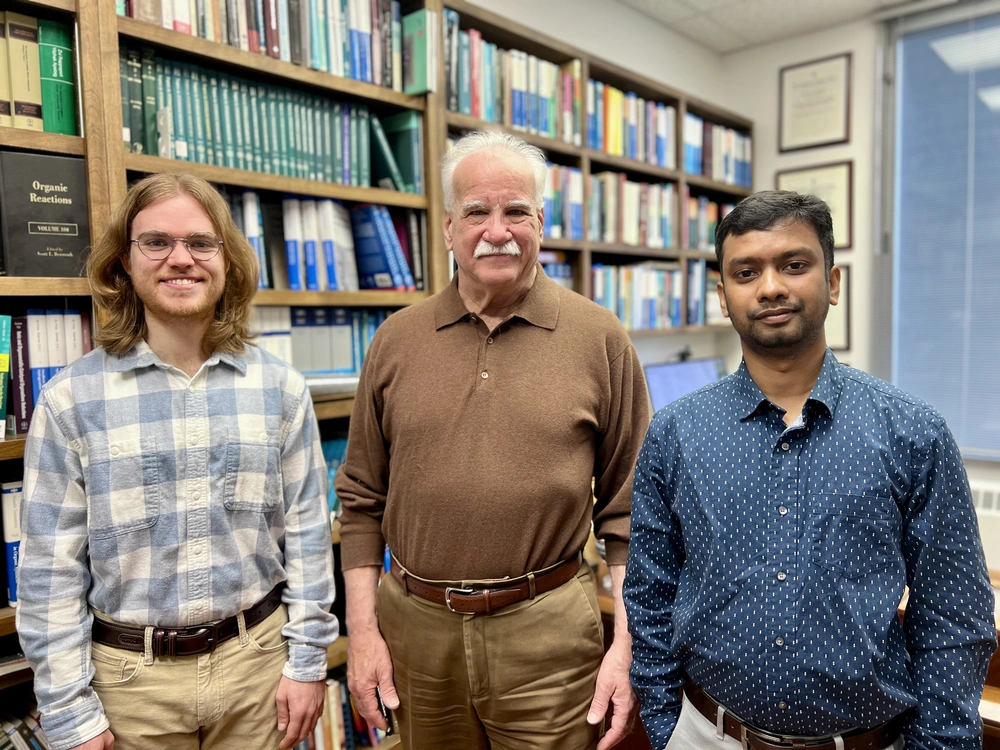
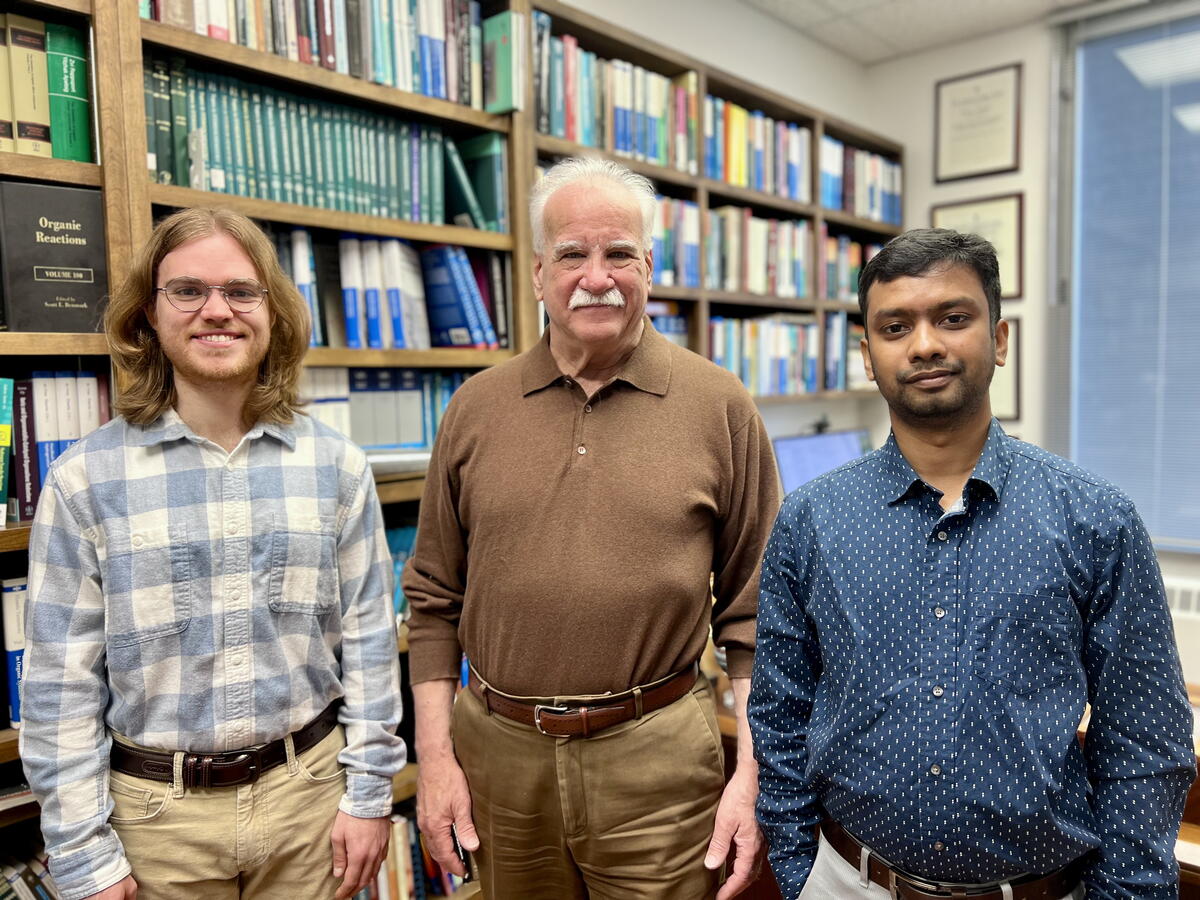
Sometimes less is more.
That’s what chemistry researchers at the University of Illinois Urbana-Champaign discovered as they were developing a new chemical reaction to produce a widely used chemical additive that gives polypropylene near-glass-like clarity and lower processing temperatures, making the plastic ideal for a variety of everyday items, from food containers to medical products, like syringes.
Polypropylene (PP) is the second-most used plastic, and the clarified form is driving a global increase in production that is expected to increase by 30 percent over the next five years, topping 100 million metric tons per year by 2030. That is also increasing demand for production of clarifying additives, which are already at 30,000 metric tons per year.
For decades, the most prevalent PP clarifying chemical has been produced in massive batches using a superstoichiometric, tin-mediated allylation, a complex, costly, and environmentally unfriendly process that results in a large amount of toxic tin byproducts.
Manufacturers have long desired a more efficient and earth-friendly way to make the clarifying chemical.
Led by Prof. Scott E. Denmark, chemistry researchers have successfully developed such a method that they describe in a paper recently published in Nature.
“It is versatile, convenient, efficient, and holds great potential for the industrial production of the clarifying agent without generating a massive amount of tin byproducts” said Dr. Tapas Adak, postdoctoral researcher.
The crucial piece to the approach is a small amount of indium metal that catalyzes the reaction.
“We only need a pinch of indium to carry out the bond formation,” said Denmark, whose research team also discovered even greater chemical significance of indium in such reactions.
“But the real societal impact,” Denmark explained, “is being able to change the way the commercial process makes this product, which is going to be so much more efficient, cost effective, and climate friendly because of the lower carbon footprint.”
Denmark had previously tried to tackle this challenge in 2008 when Milliken, the manufacturer of the most widely used PP clarifying agent, Millad® NX® 8000™, asked if he could figure out a new chemical method for their manufacturing process. In a 1,000-liter reactor, they mix a lot of ethanol, water, glucose, allyl bromide, and tin that is stirred and heated and then the tin byproduct must be neutralized and separated from the reaction mixture containing 1-allyl sorbitol (the precursor for Millad® NX® 8000™), significantly increasing the complexity and overall cost. Unreacted glucose remaining in the solution further complicates purification and ultimately reduces the overall yield of Millad® NX® 8000™.
To retool the industrial process, the crux of the chemical challenge was figuring out how to form a carbon-carbon bond (C–C) to a sugar, specifically glucose, in aqueous solution.
“Catalytically allylating unprotected saccharides in water is a difficult process and purifying the products without chromatography while maintaining excellent purity remains a significant challenge, particularly in sugar chemistry,” said Adak.
The breakthrough holds important implications for industrial applications.
“Making C–C bonds usually requires reagents and conditions not compatible with water,” said Denmark, who also explained that natural carbohydrates dissolve in water and are not compatible with most organic agents that form C–C bonds.
Nevertheless, his group came close to solving the challenge 17 years ago, developing a process that worked for five-carbon sugars, but they could not crack the code for glucose.
Fast forward to 2024, and Denmark decided to take another run at the challenge as part of the Molecule Maker Lab Institute at Illinois, a National Science Foundation funded institute that uses artificial intelligence and machine learning to develop more efficient manufacturing processes and discovery of molecules with important functions.
Dr. Adak was leading the project, trying various methods the team had designed. About six months in, he discovered a paper published by a Japanese research group led by Prof. Shu Kobayashi on a reaction that uses a pinch of indium as a catalyst to form C–C bonds to ketones in water.
“And that was the inspiration,” said Denmark, explaining that Kobayashi’s team never tried it on a sugar, because they were focusing on normal organic substrates like ketones and later hydrazones in water.
So, the team decided to try the reaction on glucose.
“And bingo, the first time we used it, it worked beautifully,” said Denmark. When asked how he reacted to the good news, he said: “Bimodal. I was so excited that he had found this, but I was disappointed that we didn’t need AI to find it.”
After a year of reaction optimization, experimental design and analysis, Dr. Adak demonstrated that indium sources were the most effective for catalytic allylation using allylBpin. With support from Travis Menard (PhD, ’24), a former grad student in the Denmark lab, and current graduate student Matthew Albritton, the team advanced the project further by incorporating allylBF₃K as the allyl donor to achieve their goal.
“AllylBF3K is commercially available, inexpensive, and it’s completely soluble in water, which is the advantage,” Albritton said.
As the team explained in Nature, “the allylation of ribose was carried out five times using the same 6.0-mg piece of indium foil that was removed from the reaction mixture, rinsed with water, and the cycle was repeated four more times. There was no notable change in yield between the first reaction (94%) and the fifth reaction (93%), and the foil lost only 0.2 mg of its original mass over the course of the five experiments.”
“We realized this is incredibly active and then the light went off. We can do this in flow,” said Denmark, explaining that rather than making a massive batch in a large reactor, the reaction could be incorporated into a flow system to continually produce 1-allyl sorbitol. That is when the team realized that they could use AI to optimize the flow process.
To do so, the team collaborated with Chemical Engineering Prof. Klavs F. Jensen at MIT to leverage the power of Bayesian optimization to streamline the continuous flow process. Dr. Adak went to MIT and worked with postdoctoral researcher Dr. Federico Florit on developing and optimizing the process.
“And that's critical because for manufacturing purposes, the footprint of the flow process is tiny compared to a 1,000-liter reactor. You exchange volume for time,” Denmark said.
Eventually, Dr. Adak and Albritton’s optimization efforts resulted in a process using indium powder to catalyze allylation of unprotected sugars in water using commercially available allylBpin and allylBF3K as nucleophiles and it works well on a variety of carbohydrates – not just glucose – and all with excellent yield.
And Dr. Adak’s work with Dr. Florit demonstrated the feasibility of producing 1-allyl sorbitol in a continuous flow system, ensuring efficiency and scalability on an industrial scale. And expertise and valuable input from Jensen and U. of I. chemistry Prof. Martin D. Burke significantly elevated the quality and impact of the project.
“This is one of the most challenging projects I have undertaken. We are glad that we are the first team to develop this reaction. This is almost 30 years old, like an unsolved problem,” Adak said, referring to the chemical challenge of forging C–C bonds with sugar molecules in water.
Their process is now under patent and Albritton has been delving into what is really going on with the highly catalytic pinch of indium, which is leading the researchers in an entirely new direction and toward another pending publication.
“It was really interesting what we found, because we didn’t know it earlier, but there are some dynamics going on with this reaction that are very unique to using sugars as substrates. It almost seems like this reaction is specifically tuned for working very well with sugars,” said Albritton, whose work has revealed additional surprises.
After running the reaction, Dr. Adak removed the indium from the solution and reran the reaction using the aqueous filtrate—observing a remarkable yield. They realized the catalyst is in the solution and now they do not need the metal for reaction. They can just use an indium salt.
“We don't know how it's getting in the solution, but that's where the active catalyst is and that's what Matthew has spent the last year ferreting out,” Denmark said. “And he used a combination of Inductively Coupled Plasma Mass Spectrometry and X-ray Photoelectron Spectroscopic analysis to prove it. We have an amazing story that he's just about ready to put on the final touches for publication.”
Albritton said they tried soluble indium salts in the beginning, but they didn’t work and assumed the indium metal worked better.
“It turns out the reason that indium metal was working so well is that only a tiny amount is leached. If you use a lot, and by a lot, I mean like only 5% of the soluble indium salt, you get moderate yields. You have to use a very, very small amount in order for it to work best,” Albritton said.
“Less is more,” Denmark added.